Microbes are a key component in healthy, organic gardens.
Among the many ways to increase beneficial microbes in the soil, a quick and effective way to do so is to add an inoculant.
What are the different types of inoculants you can use?
The most commonly used soil inoculants are mycorrhizal fungi to improve water and nutrient uptake, and Trichoderma fungi and Bacillus bacteria to prevent diseases and increase the plant’s immunity.
In this article, we will look at these 3 common types of soil inoculants, their benefits for plants, and whether you can use them together.
Let’s get started.
Quick Summary
Microbes | Characteristics |
Mycorrhizal fungi | – fungus – increases plant uptake of water and nutrients – seeks water and nutrients beyond the reach of plant roots via extensive network of hyphae |
Trichoderma | – fungus – acts as a fungicide in preventing and fighting plant diseases – increases plant immunityStimulates root growth |
Bacillus | – bacteria – forms biofilm that protects plant from diseases – increases plant immunity |
What do soil inoculants do?
Soil inoculants are microbes that are introduced to the soil usually in liquid form to help promote plant growth by increasing nutrient uptake and to prevent diseases by improving the plants’ immunity.
Seeds can be inoculated by applying microbes to them before planting so that they get to work as soon as the seeds germinate.
The most well-known soil inoculant is mycorrhizal fungi. The lesser known includes the Trichoderma fungi and the Bacillus bacteria.
1. Mycorrhizal fungi
What are mycorrhizal fungi?
Mycorrhizal fungi is any fungi that search for water and nutrients from the soil and transport them along its extensive thread-like network of hyphae called mycelium to the plant roots in exchange for a sugary solution produced by the plants in photosynthesis. Such a mutual, symbiotic relationship is known as “mycorrhizae”.
Almost all land plants partner up with mycorrhizal fungi. According to a study, around 50,000 species of fungi form mycorrhizal associations with around 250,000 plant species in all types of climates (Heijden et al., 2015).
Some plants are generalists and can partner with several species of mycorrhizal fungi, but certain plants can only partner with specific fungal species.
Types of mycorrhizae
There are 4 types of mycorrhizal fungi in nature. But the majority of fungi-plant associations are formed with the endomycorrhizal fungi (and in particular, arbuscular mycorrhizal fungi), followed by ectomycorrhizal fungi, and a very small portion with orchid mycorrhizal fungi, and ericoid mycorrhizal fungi.
Mycorrhizal type | Plants | Number of species hosting the fungus |
Arbuscular mycorrhiza (AM) | Mostly herbs, grasses and many trees | 200,000 |
Ectomycorrhiza mycorrhiza (EM) | Mostly shrubs and trees | 6,000 |
Orchid mycorrhiza | Orchids | 20,000 – 35,000 |
Ericoid mycorrhiza | Mostly members of Ericaceae | 3,900 |
Endomycorrhizae
Endomycorrhizal fungi live inside the plant roots, with their hyphae penetrating into the root cells to facilitate the transfer of nutrients between the fungi and the plant.
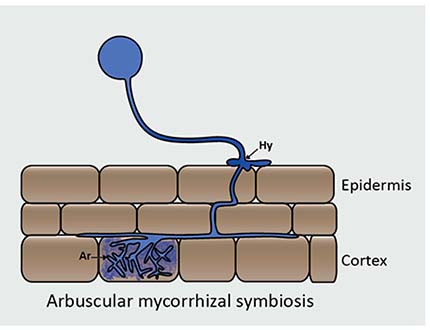
(Source: Jacott, Murray & Ridout, 2017)
Over 80% of all plants, including most agricultural crops, shrubs, grasses, and some trees, associated with endomycorrhizal fungi, specifically the Arbuscular mycorrhizal (AM) fungi (Schussler et al, 2007).
There are over 150 known species of AM fungi. The most commonly used species in soil inoculants are of the Glomus genus including:
- Glomus intraradices (also called Rhizophagus irregularis),
- Glomus mosseae,
- Glomus aggregatum.
Ectomycorrhizae
Ectomycorrhizal (ECM) fungi do not penetrate the root cells, and only live on the surface of plant roots with their hyphae forming a dense coating around the roots.
They form symbiotic relationships with around 10% of plants, particularly conifers and deciduous trees such as oak, beech, pine, and roses.
In the right season, their hyphae develop visible fruiting bodies such as mushrooms and truffles.
Examples of ECM fungi include the Boletus genus (associated with larch trees and conifers) and truffles (associated with English oak trees).
Benefits to plants
Mycorrhizal fungi play an important role in the plant’s uptake of water and key macro- and micronutrients in particular nitrogen and phosphorous.
Studies have found that AM fungi contribute up to 90% of the plant’s needs for Phosphorous (P), a key macronutrient that is difficult for plants to acquire. With mycorrhizal fungi, plants are able to access absorb phosphorous as negatively charged ions.
Studies have also known that roots that are inoculated with mycorrhizal fungi are found to absorb phosphorous 3 to 5 times more quickly than roots without mycorrhizae.
EM fungi also contribute up to 80% of the plant’s needs in Nitrogen (N) and Phosphorous (P).
They can transport moisture and nutrients from faraway places to the plant because their body is made up of an extensive network of fine thread-like hyphae (known as mycelium) which can extend up to hundreds of feet. Mycorrhizal fungi can thus improve the plant’s access to more of the soil’s resources.
With better nutrition, plants that are inoculated with mycorrhizae fungi are found to result in higher crop yields, higher tolerance to stressful soil conditions, and higher resistance against soil-borne pathogens.
Arbuscular fungi can also prevent wind and water erosion. Their long hyphae, which are covered in glomalin, act like glue and bind soil aggregates together into clumps that prevent water and nutrients in the soil from being leached away.
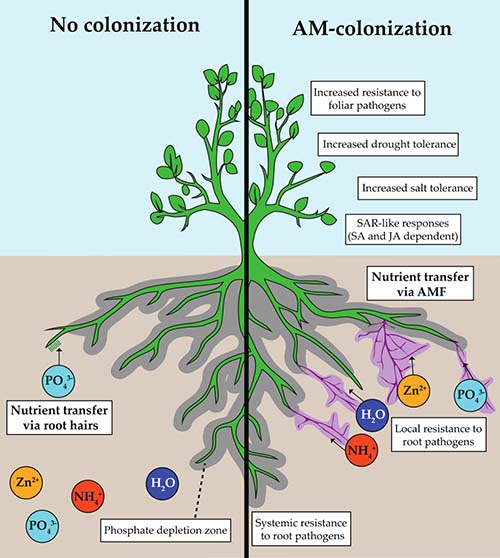
(Jacott, Murray & Ridout, 2017)
2. Trichoderma
What is Trichoderma?
Trichoderma is a genus of fungi that colonize the outer layers of plant roots or the cortex, feeding on unused nutrients on the root surface and on other soil fungi.
The fungi are microscopic, but when the hyphae come together, it forms a visible mycelium in the form of dense, white mold and eventually turns blue-green when it starts fruiting.
The optimum temperature for Trichoderma fungi is around 80 to 86 °F (27 to 30 °C), and the optimum pH of 5.5 to 7.5 (Singh et al, 2014)
Common species of Trichoderma include:
- Trichoderma harzianum
- Trichoderma viride
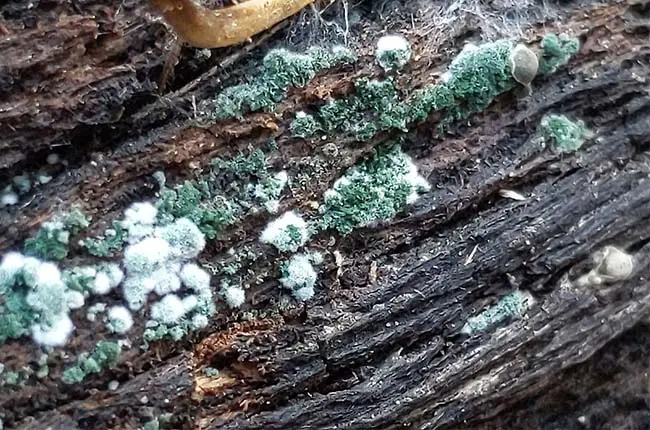
(Alan Rockefeller, CC BY 4.0, via Wikimedia Commons)
Benefits to plants
The major benefit of Trichoderma for plants is its use as a natural fungicide in controlling pathogens and stimulating root growth.
Trichoderma produces a range of antibiotics to fight off bacteria and other soil microbes, greatly reducing the chance of infecting fungal plant diseases such as fusarium root rot, wilt, and leaf rust (Harman, 2006). Because of this, it can be used on foliage as a fungicide to fight fungal diseases.
It can also prevent root disease as they colonize the rhizosphere, creating a physical barrier against pathogens from getting to the roots.
Apart from disease prevention, it can encourage the development of a deeper root system, making the plant more drought resistant and increasing its ability to pull in more nutrients. In one study, corn that is inoculated with Trichoderma was found to require 40% less nitrogen fertilizer than corn that wasn’t inoculated (Harman, 2006).
3. Bacillus
What is Bacillus?
Bacillus (full name: Bacillus amyloliquefaciens or BAA) is a species of bacteria that feeds on a sugary liquid exudated from the plant in the root zone or rhizosphere, and in turn, protects the plant from pathogens and improves its nutrient uptake.
It grows and multiplies rapidly, especially under optimal temperatures around 98 °F (37 °C) and pH levels between 5 and 7 (Gotor-Vila et al, 2017).
Benefits to plants
The main function of Bacillus bacteria in plant growth is that it eradicates pathogens and improves plant immunity.
When the bacteria lands on a root, it multiplies quickly and forms a biofilm that covers the root surface, acting as a barrier that prevents pathogens from getting through to the roots.
Studies have also shown that BAA biofilms can increase the plant’s resistance to drought Wang et al, 2019)
In addition to the biofilm, the bacteria also protect the plant by producing compounds that destroy pathogens, including the rot-causing fungi in the soil. BAA can also increase plant immunity against future stress and diseases (Siemering et al, 2016)
BAA also helps plant growth by improving nutrient uptake. They do this by producing enzymes that solubilize key nutrients like Phosphorus, Iron, and Nitrogen.
Can these microbes be used together?
The mycorrhizal fungi, Trichoderma, and Bacillus bacteria are compatible microbes and can be used together without issues. In fact, a combined use is often synergistic, producing better results than using just one type of microbe.
For example, the Arbuscular mycorrhizal fungi (AMF) improve water and nutrient absorption while the Trichoderma promotes deeper root growth and prevents diseases.
A combination of AMF and Trichoderma results in higher yield, greater root mass density, and longer bud length than using Trichoderma or AMF alone (Kakabouki et al, 2020).
A combination of AMF and the Bacillus bacteria increases water/nutrient uptake and also kills pathogens. One study found that tomatoes inoculated with BAA and AMF could use 25% less fertilizer to produce the same yield as plants that used only fertilizer and no soil inoculant (Adesemoye et al, 2009)
A combination of Trichoderma and BAA, both biocontrol agents, also produces a stronger antimicrobial effect and offers stronger protection against diseases than when used individually (Wu et al, 2018).
Conclusion
These three types of microbes offer a great boost to plant growth and disease prevention and can be used together.
The mycorrhizal fungi, mostly the Arbuscular fungi of the Glomus genus, can increase water and nutrient absorption;
Both the Trichoderma fungi and Bacillus bacteria are biocontrol agents in preventing diseases and increasing the plant’s immunity.
Take a look at our next article to find out the best products that contain these three types of microbes for your garden.
Happy gardening!
Related
8 Ways to Cultivate Beneficial Microbes (Effectively)
Here’s Why Sugar Can Fertilize Soil (With Recipe)
References
Jacott, C. N., Murray, J. D. & Ridout, C. J. (2017). Trade-Offs in Arbuscular Mycorrhizal Symbiosis: Disease Resistance, Growth Responses and Perspectives for Crop Breeding. Agronomy 7, 4: 75.
Van der Heijden, M. G. A., Martin, R. M., Selosse, M. & Sanders, I. R. (2015). Mycorrhizal ecology and evolution: the past, the present, and the future. New Phytologist.
Rinaldi, A. C., Comandini, O. & Kuyper, T. W. (2008). Ectomycorrhizal fungal diversity: separating the wheat from the chaff. Fungal Diversity, 33: 1-45.
Gotor-Vila, A., Teixidó, N., Sisquella, M., Torres, R., & Usall, J. (2017). Biological Characterization of the Biocontrol Agent Bacillus amyloliquefaciens CPA-8: The Effect of Temperature, pH and Water Activity on Growth, Susceptibility to Antibiotics and Detection of Enterotoxic Genes. Current Microbiology, 74(9), 1089–1099.
Etesami, H., Jeong, B. R., & Glick, B. R. (2021). Contribution of Arbuscular Mycorrhizal Fungi, Phosphate–Solubilizing Bacteria, and Silicon to P Uptake by Plant. Frontiers in Plant Science, 12.
Mahr, S., & University Of Wisconsin. (n.d.). Mycorrhizae. Wisconsin Horticulture.
USDA. (n.d.). Glomalin: Hiding Place for a Third of the World’s Stored Soil Carbon. United States Department of Agriculture.
Harman, G. E. (2006). Overview of Mechanisms and Uses of Trichoderma spp. Phytopathology, 96(2), 190–194.
Harman, G. E. & Cornell University. (n.d.). Trichoderma. Cornell University.
Bononi, L., Chiaramonte, J., Pansa, C., & Motinho, M. (2020). Phosphorus-solubilizing Trichoderma spp. from Amazon soils improve soybean plant growth. Nature.
Adesemoye, A. O., Torbert, H. A., & Kloepper, J. W. (2009). Plant growth-promoting rhizobacteria allow reduced application rates of chemical fertilizers. Microbial ecology, 58(4), 921–929.
Wang, D. C., Jiang, C. H., Zhang, L. N., Chen, L., Zhang, X. Y., & Guo, J. H. (2019). Biofilms Positively Contribute to Bacillus amyloliquefaciens 54-induced Drought Tolerance in Tomato Plants. International Journal of Molecular Sciences, 20(24), 6271.
Siemering, G. S. (2016). The Value of Bacillus amyloliquefaciens for Crop Production. ResearchGate.
Kakabouki, I., Tsirogiannis, D., Karydogianni, S., Folina, A., Zisi, C., Platanopoulos, E., Papadopoulos, G., Grammenos, G., & Bilalis, D. (2020). Interaction of Arbuscular Mycorrhizal Fungi and Trichoderma on Growth of Root System and on Yield of Industrial Hemp (Cannabis sativa var. ‘Uso’). Bulletin of University of Agricultural Sciences and Veterinary Medicine Cluj-Napoca. Horticulture, 77(2), 25.
Wu, Q., Ni, M., Dou, K., Tang, J., Ren, J., Yu, C., & Chen, J. (2018). Co-culture of Bacillus amyloliquefaciens ACCC11060 and Trichoderma asperellum GDFS1009 enhanced pathogen-inhibition and amino acid yield. Microbial Cell Factories, 17(1).
- Keiki Paste vs Rooting Hormone:What’s the difference? - February 4, 2024
- Top 10 Orchid Fertilizers: A Comprehensive Review (2024) - February 2, 2024
- Top 8 Soil Inoculants For Stronger Plants (2024) - February 1, 2024